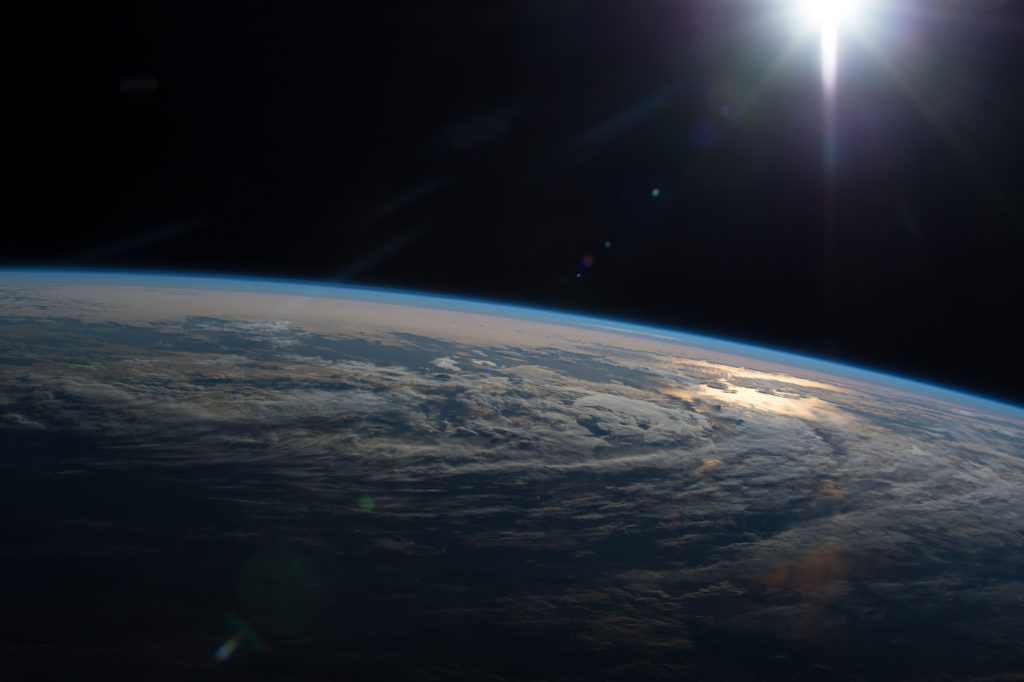
Most of the modelling of the climate system is focused on what happens inside the system – internal processes and feedbacks. However, there are some very important processes outside of the Earth’s natural climate system which need to be considered by the models. These can be things that are physically outside of the system, or things that can be determined by the modellers to force a model under prescribed climate conditions, depending on what aspects of the climate system they are simulating or studying.
Contents
Solar Orbital Forcings
Outside of the Earth’s natural climate system, the dominant forcings come from the Sun and the Earth’s relationship to it. This determines the amount of incoming radiation and its spatial and temporal distribution. These orbital cycles are also known as Milankovitch cycles, after the geophysicist and astronomer Milutin Milankovitch, who refined them back in the 1940s.
There are three main orbital cycles that describe long term changes in the way the Earth moves around the sun. Each cycle operates over long geological timescales – on the order of thousands to hundreds of thousands of years.
– Obliquity –
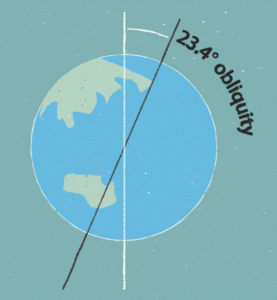
This describes the tilt of the Earth on its axis – and it shifts from 22.1 degrees to 24.5 degrees from perpendicular over a 41,000 year cycle. It’s actually this tilt that gives us the seasons. When one hemisphere points towards the Sun that hemisphere has summer, away from the sun and it has winter. At present, the Earth’s axis sits at around 23.4 degrees from perpendicular but it’s decreasing – so that the Arctic Circle (the latitude in the Northern Hemisphere where the Sun remains continuously above the horizon for 24 hours) is actually moving northward by around 15 meters per year, while the Antarctic Circle is moving south.
So why does this tilt matter? It’s only a difference of 2.4 degrees, right? Well, it doesn’t change the total amount of incoming radiation reaching the Earth, but it does affect how this radiation is distributed.
With the current decreasing angle of tilt, we can expect the high latitude summers to get colder and the mid latitude winters to get warmer. This is because the amount of radiation received in the high latitudes will decrease, just as the amount in the lower latitudes will increase. The effect is strongest at the poles, particularly in the summer and much weaker at the equator.
So, in terms of annual mean solar radiation, decreasing tilt leads to decreasing annual mean solar radiation in the high latitudes, but increasing annual mean solar radiation in the low latitudes. This difference between the poles and the equator affects the temperature gradient between these regions– which has big implications for setting up global heat distribution patterns, weather and climate. For example, in the long term, (and excluding other variables) a low angle of tilt results in cooler summers in the polar regions, allowing snow to persist year on year and potentially causing an increase in the polar ice masses.
– Precession –
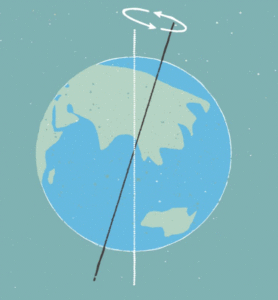
Just to complicate things further, the Earth’s axis also has a slow wobble to it – the axis itself doesn’t always point in the same direction – just like the wobble on a spinning top. This is known as the precession of the equinoxes and, when combined with the effect of the eccentricity of the orbit, it affects the hemispheric distribution of the incoming solar energy over time, influencing the intensity of the seasons. It operates over two periods of 19,000 and 21,000 years.
Summer radiation in particular is thought to be a key factor in the growth and decay of ice sheets – ie. the potential for snow melt. It’s much easier to melt an ice sheet than to build it – so a cooler summer with reduced snowmelt will trump a winter with lots of snowfall, in terms of aiding ice sheet growth.
So even though the precession of the equinoxes actually evens out on an annual basis (a warn summer one year will be compensated for by a correspondingly cold winter) the non linearities in the system highlight the importance of precession in the climate system.
– Eccentricity –
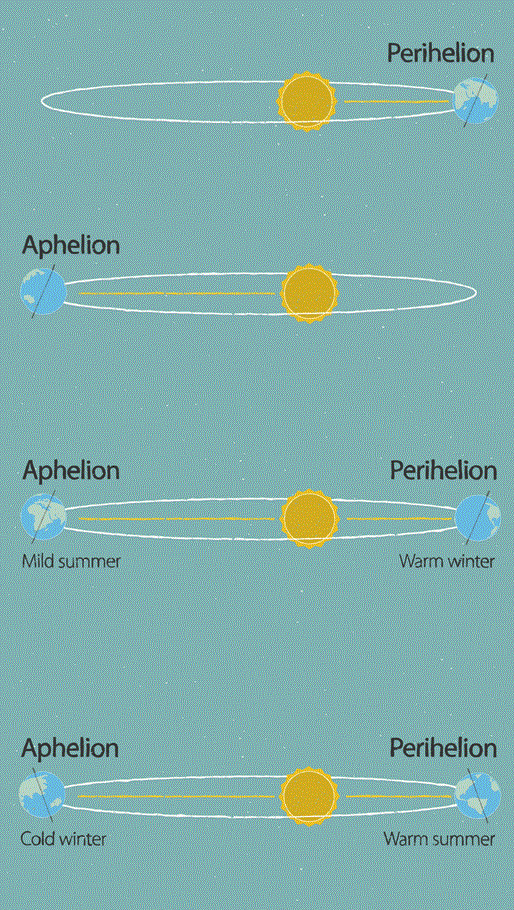
This describes the shape of the orbit of the Earth around the Sun – very slowly changing from near-circular to elliptical as the Earth’s orbit is pulled about by the gravity of other planets.
Changes in the eccentricity of the Earth’s orbit affect the total amount of incoming radiation: when the orbit is more elliptical, there is a significant difference between the amount of radiation reaching the Earth at the point where the Earth is nearest the Sun (perihelion) and when it is furthest from the Sun (aphelion).
Changes in the shape of the orbit operate over periods of around 400,000 and 100,000 years and play a role in the longer-term ice ages.
But these cycles also act together, giving a combined effect on the climate.
If the Northern Hemisphere is pointing towards the Sun (ie. summer) at the aphelion the summers will be mild and the winters will be relatively warm since they fall at the perihelion, so a relatively small seasonal contrast – particularly when eccentricity is large giving a greater difference between aphelion and perihelion.
When the opposite is true, and winter in the Norther Hemisphere falls at the aphelion and summer at the perihelion, the seasonal contrast will be much larger, with cold winters and warm summers.
But the wobble in the axis doesn’t always match neatly with the aphelion and perihelion, however, so the location of the seasons, with respect to the Earth’s position in its elliptical orbit, will change, along with their intensity.
Sunspots
The solar forcings from changes in the shape of the orbit largely work over millennial timescales – so thousands to hundreds of thousands of years. Perhaps not so relevant to a climate model looking at recent changes or near future projections then?
Well, the Sun also has an influence on much shorter timescales – the actual brightness of the sun changes. This happens on a roughly 11 year cycle and is caused by changes in sunspot activity. Sunspots are dark spots on the Sun’s surface which are surrounded by much brighter and hotter areas. The more sunspots, the more of these hot areas and the greater the luminosity of the Sun.
Sun spot activity is often associated with giant solar flares where huge amounts of solar radiation burst out from the sunspots. Take a look at the amazing NASA images of these things!
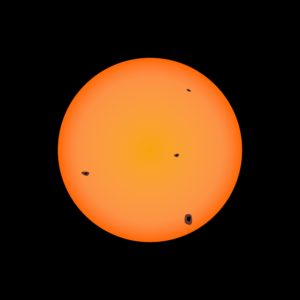
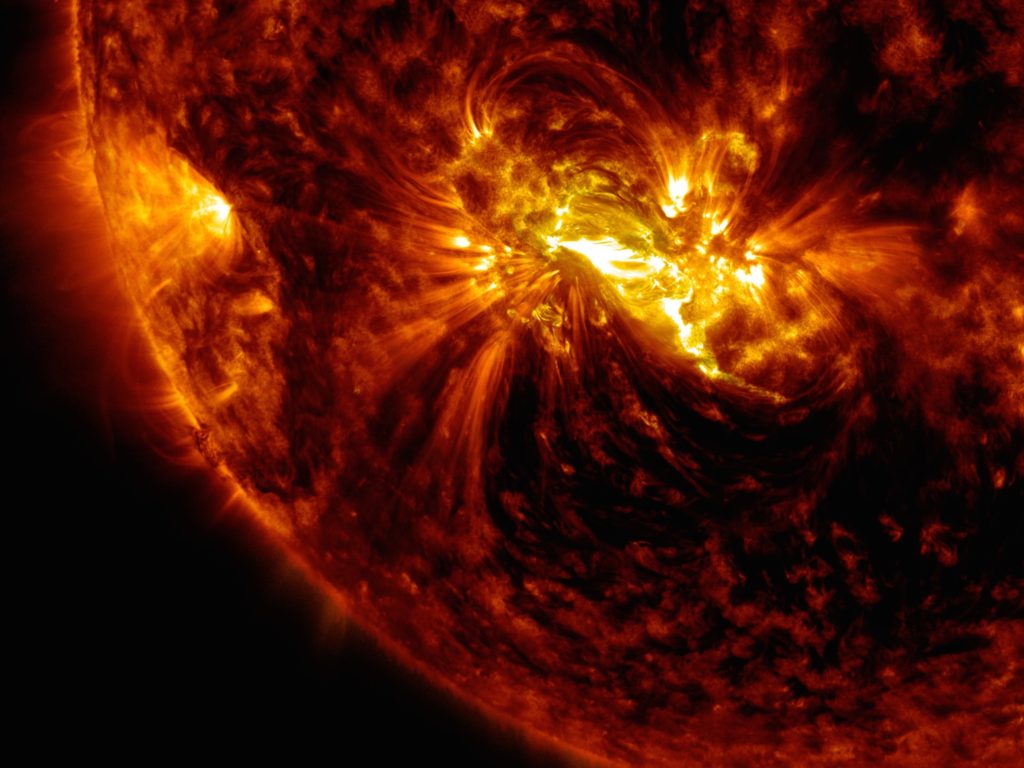
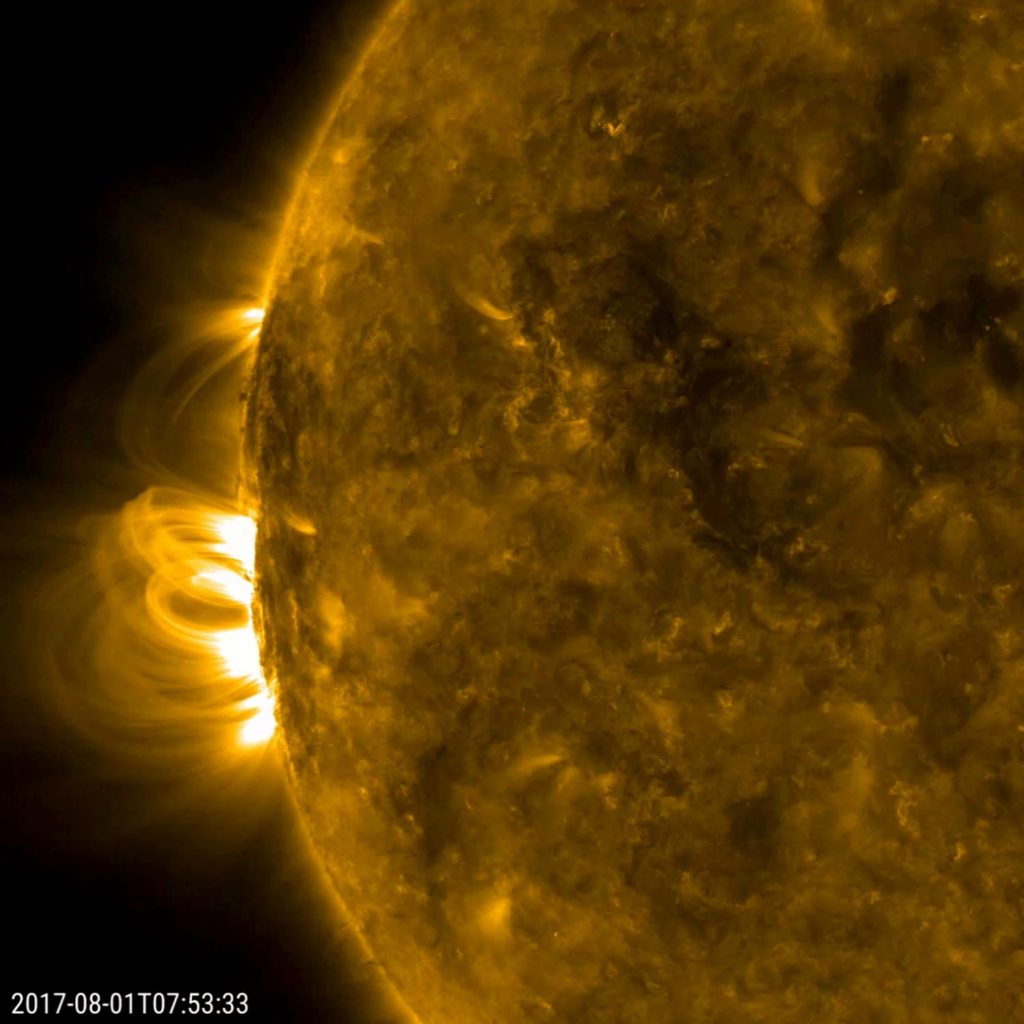
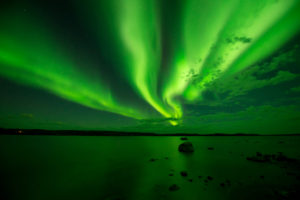
Solar flares which line up with the Earth are responsible for the beautiful polar aurora, as the Earth’s magnetosphere is bombarded with these bursts of solar radiation.
Sometimes this 11 year cycle breaks down: the Maunder Minimum during the second half of the 17th Century saw a prolonged period with very little sun spot activity. It is associated with the Little Ice Age period – a time when Europe and North America experienced exceptionally cold winters. Glaciers advanced, the Viking colonies in Greenland had to be abandoned and you could skate along the frozen River Thames in London.
Anthropogenic Greenhouse Gases
The Greenhouse Effect is a naturally occurring process – in fact it is what makes the Earth warm and habitable.
To visible light, the Earth’s atmosphere is virtually transparent, so most incoming radiation reaches easily through the atmosphere to the Earth surface, where most of the absorption occurs. This is then re-emitted from the surface in the form of infrared thermal energy – to which the atmosphere is opaque. This means that all that re-emitted energy largely ends up being absorbed by the atmosphere, causing warming. And that’s a good thing – without the naturally occurring warming effect of the atmosphere, the Earth would be just another chunk of cold, dead rock floating about in space.
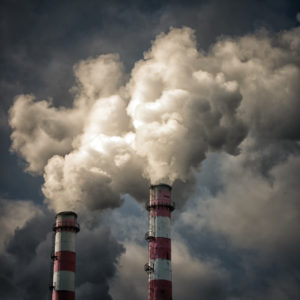
Since the onset of the Industrial period (usually given as 1750), humans have been pushing this natural process to an extreme.
In the last 150 years alone the global population has risen from around 1 billion to 7 billion, and we’re adding 200,000 new people every day. We are using 30 times the amount of energy we used back then – and at the moment, 80% of that energy comes from the burning of fossil fuels – we emit over 1000 tonnes of CO2 a minute.
Since 1750 it has become the changes in greenhouse gas (GHG) concentrations that have dominated the radiative forcing of the planet. By far the largest is CO2, but other well-mixed greenhouse gases such as CH4, N2O and the halocarbons are far from insignificant – combined, the IPCC estimates they have an equivalent radiative forcing of 2.8 Wm-2 – just have a look at the chart below.
The vast majority of the atmosphere is composed of nitrogen and oxygen but these have very little impact on the opacity of the atmosphere – it is some of the trace elements like CO2, methane (CH4), nitrous oxide (N2O) and ozone (O3), along with clouds, that have by far the most significant effect on creating an opaque atmosphere.
Some GHGs such as O3 are relatively short-lived and so tend to have a more localised effect. Others, including CO2, CH4, N2O and the halocarbons are much longer lived, remaining in the atmosphere for decades to centuries and they become relatively well mixed and homogeneous across the planet.
The chart below highlights just how much anthropogenic GHGs have some to dominate the radiative forcing of the climate. You can also see how a limited number of other factors have pushed in the other direction – the increase in aerosols has had a significant cooling effect. But nowhere near enough to offset the GHGs.
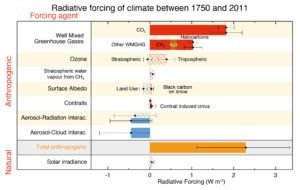
Although these GHGs physically within the climate system, their origin is man-made and so outside of the natural system. Climate modellers are looking at how this human-induced shove in the balance of the radiative forcings will effect our climate. They use GHG concentrations to force their models with prescribed conditions to see how the climate responds. What feedback loops exist in the different components to reduce or exacerbate the problem? What are the time scales of change and the regions most likely to be affected and how? The list of questions the modellers are asking is exhaustive!