Of all the components, this is the one we are the most familiar with. This is where we live, where we produce our food and go about our daily lives – and also where we have had the most visible impact on the world around us.
Land and soil ecosystems provide us with food and influence the global water and heat budgets. They also play a huge role in regulating the greenhouse gas budget of the atmosphere: photosynthesis binds carbon into living organisms, while decomposition of plant material releases carbon. Soils are huge carbon reservoirs and modellers and climate scientists are currently paying close attention to the potential role of melting permafrost and what this may mean for CO2 and methane levels in the atmosphere under future climate scenarios.
On longer and slower timescales, the bedrock composition at the Earth’s surface can also play a role in this budget – for example, the weathering of silicate and carbonate rocks can play a role in neutralising excess CO2 in the atmosphere.
Model
NorESM uses the Community Land Model (CLM) component of the Community Earth System Model (CESM) from the National Center for Atmospheric Research (NCAR).
Imagine patch of land, maybe near where you live, study or work, say, roughly 1 km by 1 km, and try to think of all of the different types of land use that occur in that area. Depending on your choice of location you might be in an urban jungle, full of high-rise buildings, roads and concrete, or a rural oasis in the mountains, with isolated cabins, snow-capped mountains and deep conifer forests. Or somewhere on the edge of a town, with buildings and fields lying side by side.
Each land use has a different environmental signature, anything from how it reflects sunlight, to how water moves across it, or even how it reacts to fires.
How that piece of land interacts with the climate system as a whole depends very much on these different types of land use.
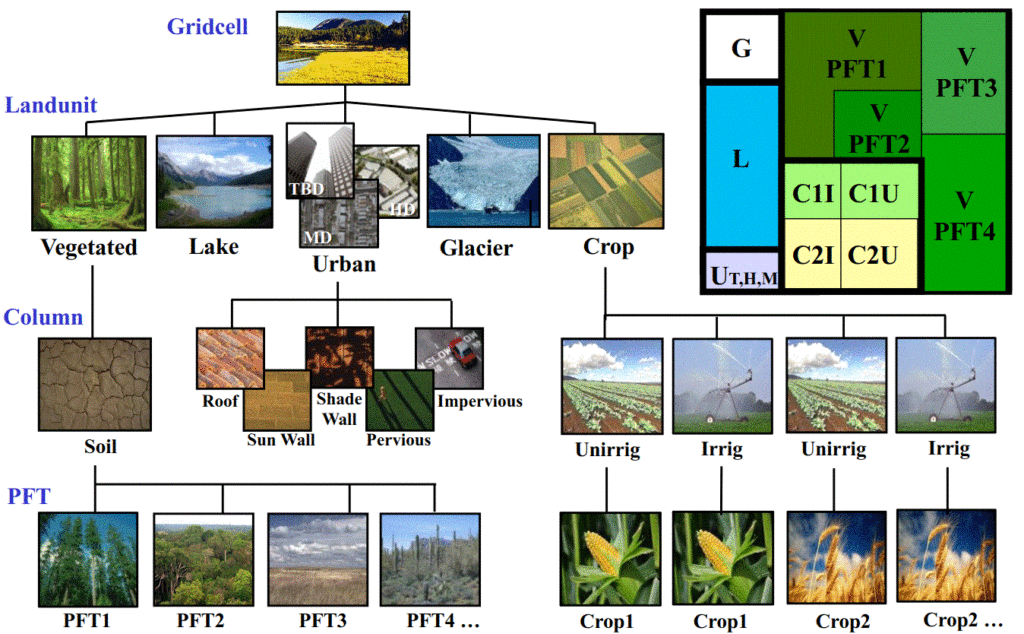
The model tries to do the equivalent of your imagined 1 x 1 km grid of land uses, but puts this into mathematical form, accounting for all the different processes and feedbacks that each type of land use has.
The model has five main land use types, which are then further sub-divided – have a look at the figure from the NCAR website above.
A grid square is broken down to represent the proportions of each land use type present. Here we see a mix of all the land use types – glacier, lake, urban, vegetated and crop.
In particular, notice how the vegetated land use is then split into a number of “PFTs” – Plant Functional Types. Each one of these plant types has its own set of properties: optical properties such as leaf reflectance; morphological properties such as canopy height, annual cycle of leaves, and root distribution; photosynthetic parameters such as leaf carbon to nitrogen ratio and leaf area, as well as it’s adaption to fire. In a similar way, the model uses a number of different crop types with their different properties, whether they are irrigated or not etc. The urban component includes information on everything from the orientation of walls for modelling the effect on the radiation budget, to what the surface is like in terms of water permeability.
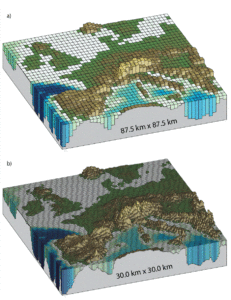
The Land model is based around two main systems: biogeophysics, which includes the surface energy fluxes and hydrology; and biogeochemistry.
Within these systems, there are also smaller models for other main component that need some extra detail, including lakes, glaciers, fire, dust emissions, river flow and biogenic volatile organic compounds
As you can see, the Land component is very detailed – there are a lot of parameters being taken into consideration!
The resolution of the Land model depends on the choice of the modeller, at 0.25°, 1° or 2° – but it takes more computing power (time and money!) to run the higher resolutions, although this can be necessary when looking at local or regional effects etc. As you can imagine, scaling up your grid square to will have quite an effect on the variations you are able to represent in your model.
Surface Energy Fluxes
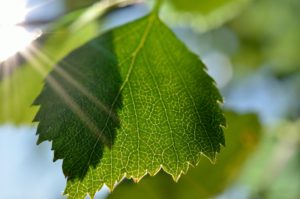
Just as our lives at the land surface are closely interlinked with the state of the atmosphere sitting directly above us, so too are the land and atmosphere components of the model. The atmosphere and land are constantly exchanging information about the local micro-meteorological conditions and those of the surface – imagine how cloud cover can affect incoming radiation to the land surface, or how temperature and wind speed can affect evaporation rates.
The land model has to constantly solve the surface energy budget for these changes, balancing the incoming and outgoing radiation as it undergoes complex processes such as absorption, reflection and diffusion. These processes can be at the small scale, such as the effect of the angle of the leaves on reflection, to the seasonal change in canopy density, or a large-scale change in land use. Dark forests, for example, have a lower albedo than a field of crops. Soil and vegetation types are therefore key information for solving the energy budget. The land model also deals with aerosol deposition onto snow, which in turn affects albedo, and dust emissions, which affect atmospheric chemistry.
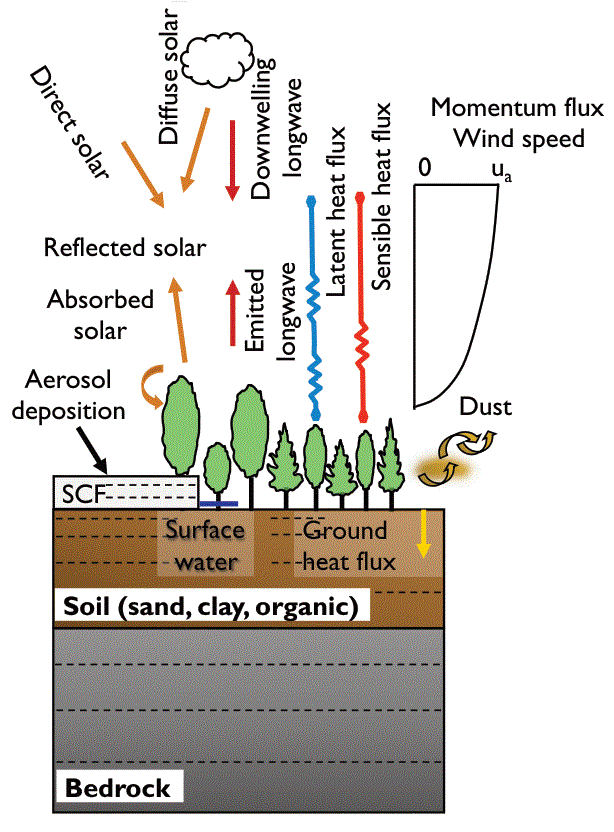
Hydrology
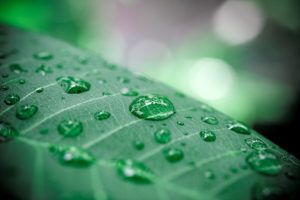
The Land model needs to understand and represent how water moves throughout the system, from the point of input from the atmosphere as forms of precipitation (rain or snow) to the output as river or groundwater flow towards the ocean, and each stage in between.
The model represents how water interacts with any vegetation within the grid cell, moving through the canopy as throughfall or stem flow. Some water will be lost back to the atmosphere via evaporation and transpiration, while much of the rest will seep down to the ground surface to either run off across the surface in streams and rivers, or infiltrate into the soil and underlying rocks and potential aquifers. The model accounts for complicated processes such as snow- and ice-melt and how evaporation and runoff vary non-linearly with soil moisture.
And obviously, vegetated and urban landscapes will have a very different hydrological system – many man-made surfaces, for example, are impervious to water, encouraging rapid surface runoff and limited infiltration in comparison to a vegetated surface with permeable soils and underlying bedrock.
A separate river transport model deals with the total runoff reaching the ocean from both the surface and the subsurface and is coupled to the rest of the land model – helping to improve land-ocean-sea-ice-atmosphere connections in the Earth system model as a whole.
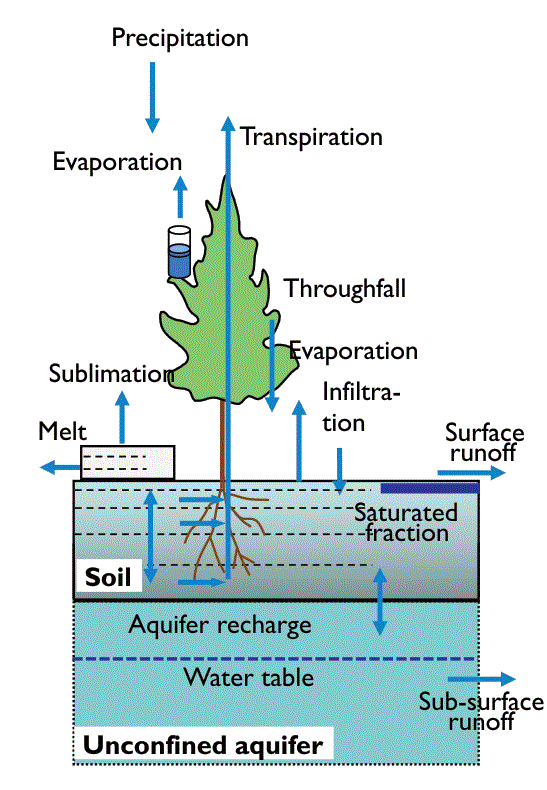
Biogeochemistry
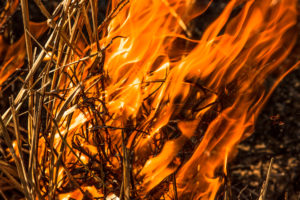
The biogeochemistry element of the land model focuses on the cycling of carbon and nitrogen within the system – from the vegetation, through the leaf litter and into the organic matter of the soil. Seasonal changes in growth and leaf litter are also included, as well as the biological mechanisms of organisms that fix carbon into the soil. The carbon cycle on land is closely tied to atmospheric CO2 levels, as well as land use.
Fire is also an important natural process. Burning is necessary for some plant species to reproduce, burning off seed casings etc, but it also aids the decomposition process, helping release nutrients back into the soil. However, fires are also significant emitters and climate scientists are interested in how biomass burning can influence climate, and indeed, how climate change may influence the occurrence and intensity of fires in the future.
The land model also contains information about biogenic volatile organic compound emissions (BVOCs). These chemicals are produced by plants and are an essential part of their growth and development, as well as defence and communication (plant to plant and plant to animal) processes. The chemicals are produced in huge volumes and have a significant effect on atmospheric chemistry and composition, particularly CO2 which is produced as the highly reactive BVOCs oxidise.
The methane cycle has also been added, allowing climate scientists to assess the role of melting permafrost in releasing the highly potent greenhouse gas into the atmosphere under future warming conditions.
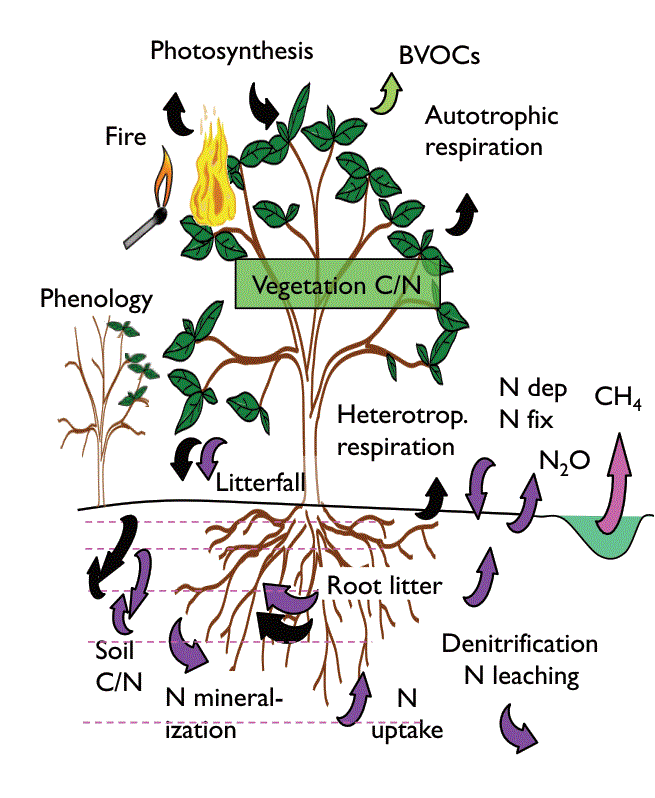
Land use changes and human impacts
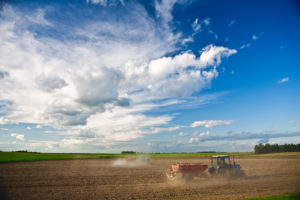
With the complexity of the land model in mind, and considering all the elements and factors it constantly assesses, just imagine the cascading effect of land use changes. The models strive to replicate this change and give us information about how this will impact things.
Land-use changes naturally over time, in parallel with the natural changes in the climate. However, humans have a much more rapid effect on land use – either directly, through forest felling, farming etc, or indirectly, through climate change related issues, such as degradation of soils, loss of sea- and land-ice etc. Often the moving biomes cannot keep up with the rapid rate of change occurring.
And our influence is irrefutable. Our activity feeds into every aspect, from large-scale land use shifts, such as urbanisation or the draining of wetlands (a natural carbon sink), to upsetting the natural nitrogen cycle through the use of artificial fertilisers, to causing extensive soil erosion through the removal of stabilising roots during deforestation. Very few areas are not directly affected by human influences, and even fewer can escape the indirect, global changes in climate that modern human activity has brought about.